Research on the relevant laser systems for laser enrichment is also currently ongoing in the United States, Russia, India, China and Iran. Third Generation Laser Uranium Enrichment Technology is likely over 5 times more energy efficient and more compact than the best centrifuges.
Nextbigfuture – It is unclear how an enforceable agreement could be reached as laser systems will become more efficient and more compact once initial commercial or weapon scale enrichment is achieved.
Long-standing efforts to develop a commercially viable laser based process for uranium enrichment, initially with atomic and later molecular isotope separation, have had limited success. This article discusses a model for a third generation of laser enrichment technology where CO2 laser light is Raman scattered to generate 16 micron photons that excite a vibrational mode in uranium-235 hexafluoride molecules within an adiabatically expanding free carrier gas jet, allowing for the partial separation of uranium isotopes by condensation repression. The SILEX (Separation of Isotopes by Laser Excitation) process being developed as part of the Global Laser Enrichment project may be one example of this separation technique. An ideal, asymmetric cascade for enriching uranium to weapon-grade levels is presented, and an analysis of the minimum laser performance requirements is included. Optimal running parameters, physical space constraints, and energy efficiency estimates are discussed. An assessment of the technical skills required is also provided. Finally, material available in an online supplement discusses possible lasers that may be utilized in such a process, and offers an introduction to dimer formation, a laser-based enrichment cascade, and a model for estimating the enrichment factor.
One third generation laser enrichment process under consideration for commercialization is known as separation of isotopes by laser excitation (SILEX). It was developed at laboratory scale by Silex Systems Ltd., an Australian company, and has been licensed to Global Laser Enrichment (GLE), a consortium comprised of General Electric (51 percent), Hitachi (25 percent), and Cameco (24 percent), for commercial development.
GLE was issued a construction and operating license for a laser enrichment plant at General Electric-Hitachi’s nuclear fuel fabrication facility in Wilmington, North Carolina, by the United States Nuclear Regulatory Commission (NRC) in 2012. GLE has stated that a decision about whether to proceed with this project will be based upon market considerations, but Silex Systems Ltd. claims key technology improvements that may lower the operating and capital costs of a commercial production facility. Other countries pursuing research related to this technology could also build plants if they believe it is cheaper to enrich uranium with lasers than with centrifuges.
Schematic of a third generation laser isotope separation unit for uranium enrichment. A high repetition rate laser tuned to excite a vibrational mode of UF cross-axially irradiates a UF/G free jet flowing from a supersonic nozzle into a low pressure chamber. The enriched product is collected with a downstream skimmer.
The importance of high isotopic selectivity requires that the UF6 gas be cooled to narrow and separate the absorption spectra. This is achieved by feeding the gas into an evacuated chamber through a nozzle to form a supersonic jet, which cools as it adiabatically expands and accelerates. Absent such cooling, thermal excitations would result in the unwanted excitation of some 238UF6 molecules due to the overlap of its vibrational absorption spectrum with 235UF6.
Supersonic speeds can be achieved when a gas passes through a converging and diverging duct, typically called a Laval nozzle.
It is estimated 300 square meters of SILEX equipment is capable of producing 32.5kg of 90% HEU annually. This estimate is almost certainly
generous.
For current Enrichment Technology Company (ETC)-supplied centrifuges to Urenco plants or American Centrifuge Plant (ACP) centrifuges, about 50 kWh/SWU is required. The 12.7 kWh/SWU (or 1.29 kWh/SWU for ten meters SILEX) estimate is low considering that 10.2 μm light must be Raman-shifted, and only 55 percent energy efficiency is claimed in the shift to 16 μm. In addition, the electro-optical efficiency of the TEA CO2 laser is only 15 percent to 26 percent prior to Raman-shifting. These inefficiencies change the energy consumed to 88.8−154 kWh/SWU for one meter and 9.02−15.6 kWh/SWU for ten.
A 5000 SWU SILEX-type plant operating at 10 kWh/SWU would consume as much energy as a grocery store in the United States that was only 100 square meters in size.
With some laser enrichment and cascade designs the SILEX efficiency could be greater than state of the art centrifuges by a factor of 5, and possibly much higher.
SOURCES – Science and Global Security
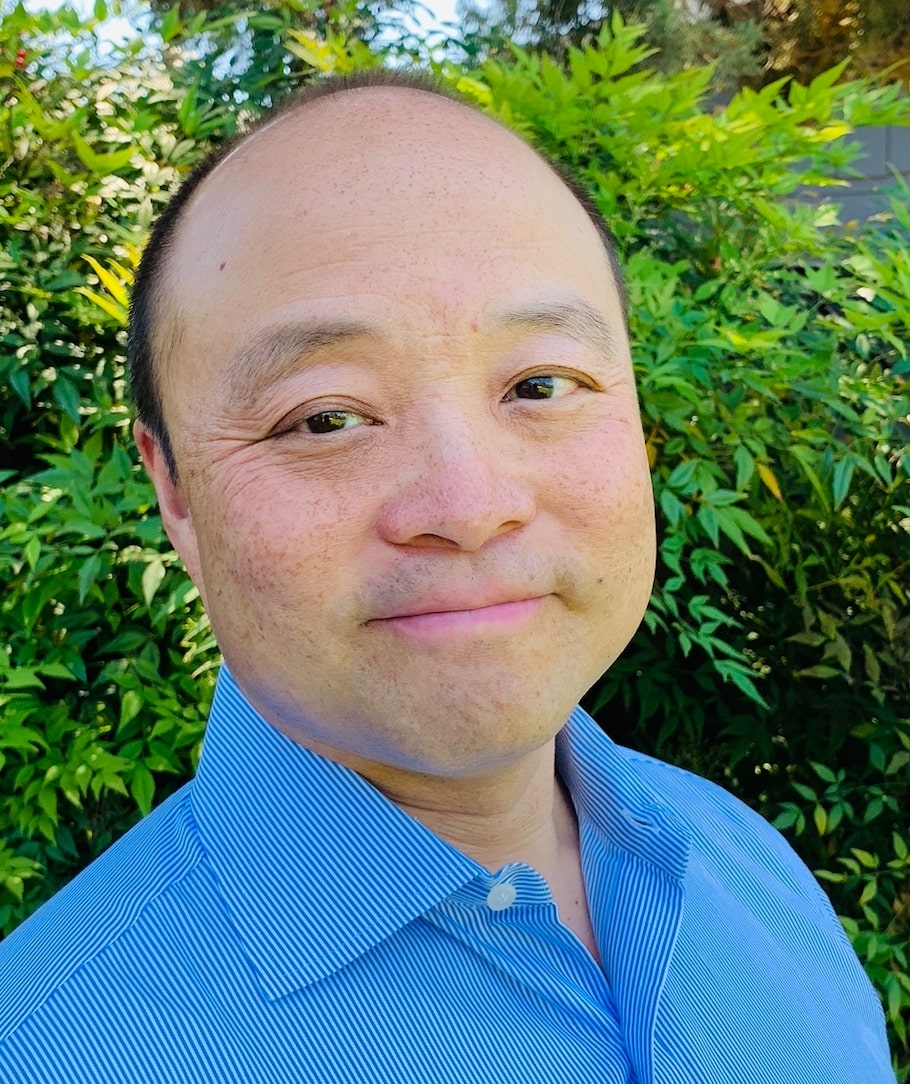
Brian Wang is a Futurist Thought Leader and a popular Science blogger with 1 million readers per month. His blog Nextbigfuture.com is ranked #1 Science News Blog. It covers many disruptive technology and trends including Space, Robotics, Artificial Intelligence, Medicine, Anti-aging Biotechnology, and Nanotechnology.
Known for identifying cutting edge technologies, he is currently a Co-Founder of a startup and fundraiser for high potential early-stage companies. He is the Head of Research for Allocations for deep technology investments and an Angel Investor at Space Angels.
A frequent speaker at corporations, he has been a TEDx speaker, a Singularity University speaker and guest at numerous interviews for radio and podcasts. He is open to public speaking and advising engagements.