MIT engineers have developed a fuel cell that runs on the same sugar that powers human cells: glucose. This glucose fuel cell could be used to drive highly efficient brain implants of the future, which could help paralyzed patients move their arms and legs again.
The fuel cell, described in the June 12 edition of the journal PLoS ONE, strips electrons from glucose molecules to create a small electric current. The researchers, led by Rahul Sarpeshkar, an associate professor of electrical engineering and computer science at MIT, fabricated the fuel cell on a silicon chip, allowing it to be integrated with other circuits that would be needed for a brain implant.
The new twist to the MIT fuel cell described in PLoS ONE is that it is fabricated from silicon, using the same technology used to make semiconductor electronic chips. The fuel cell has no biological components: It consists of a platinum catalyst that strips electrons from glucose, mimicking the activity of cellular enzymes that break down glucose to generate ATP, the cell’s energy currency. (Platinum has a proven record of long-term biocompatibility within the body.) So far, the fuel cell can generate up to hundreds of microwatts — enough to power an ultra-low-power and clinically useful neural implant.
“It will be a few more years into the future before you see people with spinal-cord injuries receive such implantable systems in the context of standard medical care, but those are the sorts of devices you could envision powering from a glucose-based fuel cell,” says Benjamin Rapoport, a former graduate student in the Sarpeshkar lab and the first author on the new MIT study.
Rapoport calculated that in theory, the glucose fuel cell could get all the sugar it needs from the cerebrospinal fluid (CSF) that bathes the brain and protects it from banging into the skull.
Power Extraction from Cerebrospinal Fluid by an Implantable Glucose Fuel Cell. Conceptual schematic design for a system that harvests power from the cerebrospinal fluid, showing a plausible site of implantation within the subarachnoid space. The inset at right is a micrograph of one prototype, showing the metal layers of the anode (central electrode) and cathode contact (outer ring) patterned on a silicon wafer. Image Credit: Meninges and Vascular Anatomy courtesy of the Central Nervous System Visual Perspectives Project, Karolinska Institutet and Stanford University.
PlosOne – A Glucose Fuel Cell for Implantable Brain–Machine Interfaces
Sarpeshkar’s group has worked on all aspects of implantable brain-machine interfaces and neural prosthetics, including recording from nerves, stimulating nerves, decoding nerve signals and communicating wirelessly with implants. One such neural prosthetic is designed to record electrical activity from hundreds of neurons in the brain’s motor cortex, which is responsible for controlling movement. That data is amplified and converted into a digital signal so that computers — or in the Sarpeshkar team’s work, brain-implanted microchips — can analyze it and determine which patterns of brain activity produce movement.
Although he has just begun working on bringing ultra-low-power and medical technology to market, he cautions that glucose-powered implantable medical devices are still many years away.
Abstract – We have developed an implantable fuel cell that generates power through glucose oxidation, producing steady-state power 3.4 microwatts per square centimeter and up to 180 microwats per square centimeter of peak power. The fuel cell is manufactured using a novel approach, employing semiconductor fabrication techniques, and is therefore well suited for manufacture together with integrated circuits on a single silicon wafer. Thus, it can help enable implantable microelectronic systems with long-lifetime power sources that harvest energy from their surrounds. The fuel reactions are mediated by robust, solid state catalysts. Glucose is oxidized at the nanostructured surface of an activated platinum anode. Oxygen is reduced to water at the surface of a self-assembled network of single-walled carbon nanotubes, embedded in a Nafion film that forms the cathode and is exposed to the biological environment. The catalytic electrodes are separated by a Nafion membrane. The availability of fuel cell reactants, oxygen and glucose, only as a mixture in the physiologic environment, has traditionally posed a design challenge: Net current production requires oxidation and reduction to occur separately and selectively at the anode and cathode, respectively, to prevent electrochemical short circuits. Our fuel cell is configured in a half-open geometry that shields the anode while exposing the cathode, resulting in an oxygen gradient that strongly favors oxygen reduction at the cathode. Glucose reaches the shielded anode by diffusing through the nanotube mesh, which does not catalyze glucose oxidation, and the Nafion layers, which are permeable to small neutral and cationic species. We demonstrate computationally that the natural recirculation of cerebrospinal fluid around the human brain theoretically permits glucose energy harvesting at a rate on the order of at least 1 mW with no adverse physiologic effects. Low-power brain–machine interfaces can thus potentially benefit from having their implanted units powered or recharged by glucose fuel cells.
Substrates, masks, and lithography.
Electrodes, separator membranes, wire traces, and metal contacts were all patterned using conventional photolithography, with transparency masks printed to 2 µm feature-size tolerance (Infinite Graphics, Minneapolis, Minnesota). As substrates, we used 500 nm surface layers of silicon dioxide on conventional, 150-millimeter-diameter (6-inch) silicon wafers. To promote platinum adhesion, we deposited a 2 nm coat of titanium nitride on the oxide by evaporation.
If you liked this article, please give it a quick review on ycombinator or StumbleUpon. Thanks
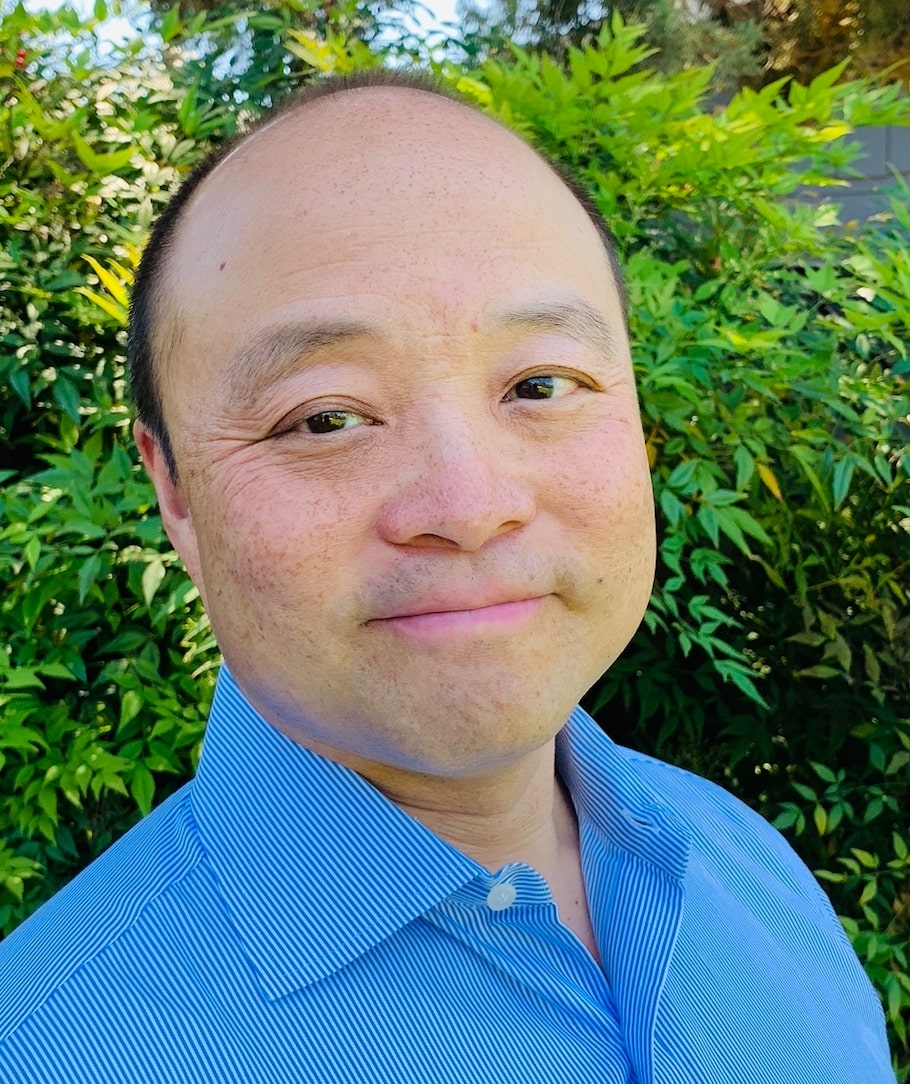
Brian Wang is a Futurist Thought Leader and a popular Science blogger with 1 million readers per month. His blog Nextbigfuture.com is ranked #1 Science News Blog. It covers many disruptive technology and trends including Space, Robotics, Artificial Intelligence, Medicine, Anti-aging Biotechnology, and Nanotechnology.
Known for identifying cutting edge technologies, he is currently a Co-Founder of a startup and fundraiser for high potential early-stage companies. He is the Head of Research for Allocations for deep technology investments and an Angel Investor at Space Angels.
A frequent speaker at corporations, he has been a TEDx speaker, a Singularity University speaker and guest at numerous interviews for radio and podcasts. He is open to public speaking and advising engagements.